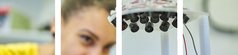
Pretty flat – from thin layers to 2D materials
Two-dimensional magnetic semiconductors
Within the scope of its ongoing theoretical research into novel two-dimensional materials, Professor Peter Kratzer’s research group has recently begun to examine materials with magnetic properties. Chromium(III) iodide CrI3 is a well-known member of this class of materials. A stack of atomically thin single layers that are magnetised in the same or different directions could function as an extremely compact, magnetic store of information in future. Due to variable electrical resistance, a current flowing perpendicular to the layers can be used to read stored information. Using a special calculation process, members of Professor Peter Kratzer’s research group have successfully modelled the magnetic interaction between the layers and the ratio of resistance with identical and divergent magnetisation of the atomic layers in line with the experimental data. Their results may provide a basis for identifying alternative candidates for envisioned applications, i.e., materials with an altered chemical composition and, as such, enhanced magnetic functionality.
Nanoscale materials for energy conversion
Professor Rossitza Pentcheva’s research group uses high-performance computing systems for nonparametric quantum-mechanic modelling of novel materials used in electronic components and energy conversion.
Within the scope of CRC/TRR 80, researchers are studying the occurrence of new electronic phases at transition metal oxide interfaces. This includes topologically non-trivial states in oxide superlattices with honeycomb patterns and ultra-thin films whose properties change drastically under extreme tension, e.g., from a ferromagnetic metal to an antiferromagnetic insulator. Further research focuses on improving thermoelectrical properties by taking advantage of reduced dimension, e.g., in oxide heterostructures. This has led to a European patent. In CRC/TRR 247, researchers optimise anode materials for water splitting by precisely modifying their structural motifs, chemical composition and defects. CRC 1242 focuses on modelling the propagation of laser pulses through metal-insulator interfaces on an ultra-short time scale and the accurate description of spectroscopic properties through many-body theory. Last but not least, the newly established CRC/TRR 270 investigates how a combination of magnetism and lattices can increase the efficiency of magnetocaloric materials.
Thin materials: graphene
Ultra-thin materials are en vogue. Right after their discovery, these materials with a thickness of only few atomic layers mostly attracted the attention of basic research. Recently, however, application-oriented research has become increasingly interested in them, too. The European Union recognised this potential at an early stage and established the Graphene Flagship in 2013. Within the flagship, a team of researchers from the Delft University of Technology (Professor Peter Steeneken’s research group), the Université Basse Normandie and the University of Duisburg-Essen (Professor Marika Schleberger’s research group) has successfully built a graphene-based pressure sensor that can detect gases with extremely high precision. In this process, a small gas reservoir is sealed with a graphene layer that is less than a nanometre thick and perforated with defined pores. What is special about this sensor is that it does not rely on chemical reactions. Instead, detection is based on the permeation time of gases through the porous graphene membrane, which simultaneously functions as a gas pump and a pressure sensor. The idea behind it is simple: light gases are faster, so they escape the reservoir sealed by the membrane more quickly than heavy gases do. This system has a range of advantages over conventional sensors: the sensor is extremely small, fast and energy-efficient yet highly sensitive.
Next-generation solar cells
Material efficiency and more effective light conversion are key topics in photovoltaics. Professor Martina Schmid’s research group develops next-generation thin-film solar cells. They are ultra-small or ultra-thin but still harvest sunlight efficiently when combined with optical concepts. In particular, the researchers have made important progress in their work on ultra-thin solar cells with an absorber made of Cu(In,Ga)Se2 applied to a transparent substrate. They have achieved an efficiency of nearly 13% for an absorber layer thickness of less than 500 nm, which trumps results achieved by other groups in the past. Additional improvements are expected as a result of combining the existing system with nano- and micro-optical concepts for targeted light harvesting. Ultra-thin solar cells with transparent back contacts can be used for a wide range of purposes in high-efficiency concepts and in the aesthetic aspect of building integration.
The world’s thinnest films
Besides ultra-fast structural dynamics at solid-state surfaces, Professor Michael Horn-von Hoegen’s research group studies the growth of the world’s thinnest films. The researchers produce 2D materials, layers that are a single atom thick and exhibit completely novel properties compared to volume. They were able to decipher a new growth mode for monolayer boron or ‘borophene’, which is just as relevant to other materials: boron atoms are dissolved in a metallic substrate at a high temperature following the decomposition of a boronic precursor. They segregate to the surface while cooling and form a perfect monolayer of borone. The boron functions as a surfactant (‘surface active agent’) and smoothes the metallic substrate to grow as a perfect layer.