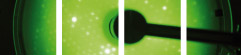
Physics
Nanostructures
One of the themes of the Lorke research group is the optical and electronic properties of nanostructures. As the name suggests, nanostructures have dimensions of just a few nanometres – millionths of a millimetre. They are fabricated either chemically (extremely finely distributed particles in smoke) or physically (the tiniest droplets in a spray mist). A third method is lithography, in which the required structures are cut out of various materials with nanometre precision. This is possible on the “nanoworkbench”, which can be used to define virtually any shape with highly focused beams of electrons and ions. This instrument has been used to cut the thinnest material in the world into shape. That material is “graphene”, comprising just a single layer of carbon atoms, which is suspended like a hammock between two metal strips. The entire structure is one hundredth of the diameter of a hair in size. The goal is to use this technique to manufacture functional electronic components such as transistors and rectifiers.
Self-organised nanostructures are also being investigated for their potential in novel components. For example, members of the research group have been able to enter single electrons in quantum dots and read out the number of electrons electrically in just three nanoseconds (0.000’000’003 seconds) – an initial step towards creating a quantum dot memory.
A surprising observation was made on nanoparticles made of a silicon-iron compound when it was found that they can be used to make a kind of battery. The particles simply need to be introduced between two electrodes to which a voltage can be applied. The exact mechanism has not been deciphered yet, but the Lorke research group has nevertheless been able to show that water (and other common solvents) in the ambient air plays an important role. Given the potential applications for energy storage and gas detection in air, a patent has been filed for this chance discovery.
That is not all nanoparticles can do, however: In collaboration with scientists from Electrical Engineering at the UDE and the University of Minnesota, zinc-oxide particles were manufactured which have particularly good illuminating properties. This makes them interesting as potential energy-efficient light-emitting diodes, which additionally work without scarce elements such as gallium. Further international collaboration in the field of optics took place with the Japanese University of Tsukuba. On microscopic spheres of a novel polymer, the researchers discovered what are known as whispering gallery modes, in which light travels around the inside of the spheres as if on a racing track. This is evidence of the high optical quality of the polymer spheres and opens up potential for laser and photonic crystals made of organic materials.
The research group of Prof. Marika Schleberger is concerned with how ultrathin two-dimensional materials can be manufactured and specifically modified. Initially their interest was limited to single-atom carbon layers (“graphene”); now, however, they are exploring other materials, including hexagonal boron nitride (“white graphene”) or molybdenum disulfide (MoS2), a known lubricant. An important tool in manipulating the material properties is irradiation with energetic ions. The experiments conducted in the Schleberger research group show, e.g. that transistors made of graphene withstand substantially higher levels of radiation exposure than transistors based on MoS2. They are currently examining what minimum level of energy input is sufficient to damage such ultrathin films. The results will enable the researchers to determine whether the novel materials are suitable for future electronics components which are exposed to extreme levels of ionising radiation, e.g. in space or in fusion power plants.
The destructive effects of particle radiation can also be put to constructive use. The research group has shown that it is possible to burn tiny pores into the aforementioned materials under the right conditions. The pore size is typically within the nanometre range, which suggests possible applications as filter membranes for minute particles, such as viruses. However, there is a range of problems to be solved before that will become possible. For example, although graphene is a good hundred times more tear-resistant than steel, that only applies at a comparable thickness. And because graphene is exactly a single atomic layer thick, it should only take minimal loads to destroy a graphene filter. The current research work in cooperation with other researchers therefore aims to determine the exact conditions for creating the necessary pore sizes and develop a suitable carrier material.